Physical design software: the only moats in SaaS
An overview of the history, market, and trends in CAD, simulation, and EDA software
When we look back at the history of software, we see that nearly every software category has seen waves of new companies ride technology shifts to unseat incumbents. The cloud laid the path for much of this disruption. It fundamentally lowered the barrier of entry to software development. Companies could now build software with a credit card instead of a data center. Nearly every industry in software saw “cloud-native” businesses rise up and compete with incumbents.Â
One industry stands out as an exception: physical design software.Â
The CAD, EDA, and simulation providers are more dominant now than ever.Â
This article will examine the history of physical design software, explain why the leaders have remained dominant over time, provide an overview of the current technology and markets, and offer commentary on what the future of this industry could look like.Â
Before diving in, here’s the current landscape of the physical design process, more details later:
‍

‍
A history of physical design software
The history of physical design software can broadly be broken down into two parts: the industry’s early history and the rise of the “modern” design companies.Â
Much of this section was informed by ”The Engineering Design Revolution—A History of CAD” by Shapr3D.Â
‍
The early history of design software
Before the 1960s, physical systems were designed and drafted by hand.
‍

‍
Like many technical trends, the CAD industry began within large companies. In 1957, Patrick Hanratty (often called the father of CAD) created Design Automated by Computer (DAC) in GM’s Research Labs. By the mid-1960s, large manufacturing companies had begun to realize the value of computer-based design. Most notably, automotive companies like Renault and Ford focused on mathematically modeling complex surfaces. The Renault systems would become Dassault Systemes, one of the four major general CAD companies today.
Soon after, though, the industry saw the first commercial systems from five primary companies: Applicon, ComputerVision, Auto-trol, Calma, and M&S Computing (Integraph). These companies mainly were hardware manufacturers who developed software to help sell their hardware. Each machine sold for about $150,000 in 1972. Ansys would be founded around this time as well for simulating engineering scenarios.
This 5-company oligopoly would strengthen until the rise of the workstation and the PC.
‍
The rise of “modern” design software
The decade of the 1980s would shake up the existing oligopoly and cement the industry’s structure for the next (*checks calendar*) 40 years.Â
At the beginning of the decade, each machine cost ~$300k and was operated by CAD engineers. The rise of the engineering workstation (from companies like Apollo) led to the separation of hardware and software. Shortly after, the PC was released as well, although it took time for the performance of PC semiconductors to support CAD software. This opened the door for early software companies to only develop software and not worry about hardware development.
From 1981 to 1986, six of today’s oligopolistic design companies were founded: Mentor Graphics (1981), Autodesk (1982), Cadence (1983), Bentley Systems (1984), PTC (1985), and Synopsys (1986). These companies made design software specifically for workstations and/or PCs, creating a cheaper and more flexible alternative to the incumbents.
The PC CAD vendors also made a savvy move (one that Clay Christensen would be proud of):
‍
This provided a fundamentally disruptive business profile that the incumbents couldn’t keep up with.Â
From the mid-1990s to today, the industry has consolidated through acquisitions and product expansion. The general CAD providers now have a four-company oligopoly with Autodesk, Dassault, PTC, and Siemens (whose CAD/PLM division would be formed through acquisitions in the 2000s). EDA providers have consolidated to Synopsys, Cadence, and Siemens EDA. With Synopsys’ acquisition of Ansys, the industry will further consolidate.Â
If this ending of history feels rather abrupt, that’s because it is. The industry hasn’t seen major changes since the 1980s. Companies have switched pricing models and have started to migrate to cloud-based versions of products, but the industry structure has remained mostly constant.
This leaves us in an oligopoly industry, where we need to ask, “Is it possible for this to change, and what would need to happen to drive that change?”
‍
Why haven’t these companies been disrupted?
It’s important to take a step back and answer the most interesting question driving this industry—about the lack of disruption. The answer is essentially a case study on what software moats can look like. There are four driving forces here:
- Technical complexity: This is some of the most complex software in the world. EDA software, for example, has millions of lines of code for each tool. Startups can spend 5+ years just hitting feature parity for CAD platforms. So, while it’s hard to build these tools that are just as good, it’s even harder to build tools that are better.Â
- Switching costs and risks: Do companies want to risk moving their decades of IP and retraining employees? The complexity of the software means moving tools requires significant retraining time, meaning less productivity time and potential downtime. Plus, if design flaws result from switching software, the costs are much higher than software defects.Â
- The GTM problem: Most Fortune 100 hardware and adjacent companies use these tools and have little reason to move. Mid-market and SMBs have Autodesk as a standard, which is taught in schools, and engineers spend years getting certified in it. These are industry defaults. There aren’t many scaled consumer use cases, so the bottom of the market doesn’t provide much value. This means there’s no good place for a company to start selling.Â
- Hardware engineer ≠software engineer: While hardware engineers have some of the most expensive software licenses — CAD, simulation and modeling platforms — they typically don’t have purchasing power to drive tool changes. The cultures and processes within hardware organizations are vastly different from those of software, leading to lower novel tech absorption rates and a difficult bottoms-up GTM for new products.Â
All of this leads to the “inertia” of software businesses, meaning an alternate product has to be a few orders of magnitude better for a company to switch software products. As of yet, we haven’t seen a large enough performance gain to justify companies switching their critical software stack at a large scale.
‍
An overview of the physical design process
The evolution of the physical design software industry has been characterized by the consolidation around a few dominant players. Over the past four decades, companies like Autodesk, Cadence, and Synopsys have expanded their technological capabilities and also absorbed competitors through strategic acquisitions, reinforcing their market positions.
Here’s an image breaking down the physical design process in all its phases:
‍

1. Requirements gathering and conceptual design
The first step in design is gathering requirements from internal stakeholders, suppliers, and customers. A project manager coordinates interactions with all these people and ensures that a design/component is being developed according to specifications.Â
‍
Then, an industrial designer will create a conceptual design, get feedback from stakeholders, revise it, and pass it on to the engineering team. For this step, they’ll typically use surface modeling tools like Autodesk Alias or Rhino3D.
‍‍
2. Detailed design
An engineering team will then refer back to the requirements and start to design the actual component/system. The steps will go from system design (“I need an engine of X size”) to subsystem design (“I need x amount of cylinders”), to component design (the parts that make up cylinders). For mechanical systems, generic CAD tools will typically be used.
Many industries, however, need domain-specific design tools. Some examples include EDA tools for chip design, PCB tools for circuit boards, BIM tools for building construction, specific tools for roads/bridges/tunnels/water, and manufacturing plant design.Â
‍
3. Simulation
After the system has been designed in detail, the system needs to be simulated as close to real-world scenarios as possible. This includes techniques like finite element analysis for load testing and computational fluid dynamics for liquid/gas flows. Any failures need to be redesigned and retested. Ansys is the leading provider in this space.Â
‍
4. Design for Manufacturing & Verification & Validation
Essentially, 3D CAD tools can create any shape in existence. But we can’t manufacture any shape in existence. DFM is the process of analyzing what each manufacturing step is capable of: what materials are available, are they high-volume, does it have to be metal, can the shape be simplified, and how will it be manufactured—injection molding or casting?
Verification and validation are the final testing steps, which are especially important in chip design. The actual performance of the chip/product needs to be tested as thoroughly as possible, as any manufacturing flaws will result in millions of dollars of waste. Any flaws need a root cause analysis, a fix, and a retest.
This is the most manual step of the design process right now. For large projects, an experienced designer is typically required at this stage.Â
‍
5. Project lifecycle management
PLM tools manage the data and components on the back end throughout this process. The first part is managing parts in a tree-like fashion, tracking which components are in each system and changes to any process. The second piece is project data management, managing data such as CAD files, bills of materials, and version controls.
An overview of the physical design software market
You can divide segments of this market into the four general CAD providers, domain-specific providers, and challengers.Â
‍

‍
The CAD oligopoly: Autodesk, Dassault, Siemens, and PTC
These four companies offer a similar breadth of products with different focuses on verticals and market segments. They all offer CAD, PLM, and a host of other design software.Â
Autodesk started with AutoCAD, focusing on individual users and less so on enterprises, and they’ve maintained that position. The largest part of their business is in the architecture, engineering, and construction “AEC” industry with BIM software (Revit, AutoCAD Civil), which is almost 2x larger in revenue than their CAD business. They dominate the mid-market and SMBs for CAD software (AutoCAD, Inventor). They also offer an incredible breadth of products: PCB design, construction project management software, water system design, construction payroll, and bid management, so they’re expanding both in engineering and construction software and in design software.Â
Dassault is the oldest of the four players and the slowest growing. Their initial niche was for automotive and aerospace companies, and they are still the market leader in those spaces. Their enterprise offering is CATIA, and their mid-market offering is Solidworks, an industry standard. Solidworks is the most popular CAD platform for students and universities.
Siemens is a massive industrial company, and they’ve continued to grow their industrial software business. Their integration with PLCs (computers for manufacturing lines) provides them with a hardware/software integration and a distribution network advantage. Their Siemens NX software is an enterprise CAD product utilized by companies like Apple and SpaceX, while Solid Edge is a mid-market product. Siemens has the largest PLM business of the four, and it’s common to have Siemens PLM with CAD from another provider.
Finally, PTC is the smallest of the four. They focus on the manufacturing segment and made a push in the 2010s to integrate AR/VR and factory analytics into their platform. Those efforts haven’t been too successful and likely are a product of making acquisitions too early before the technology was ready.Â
We can see their market segmentation here:
‍
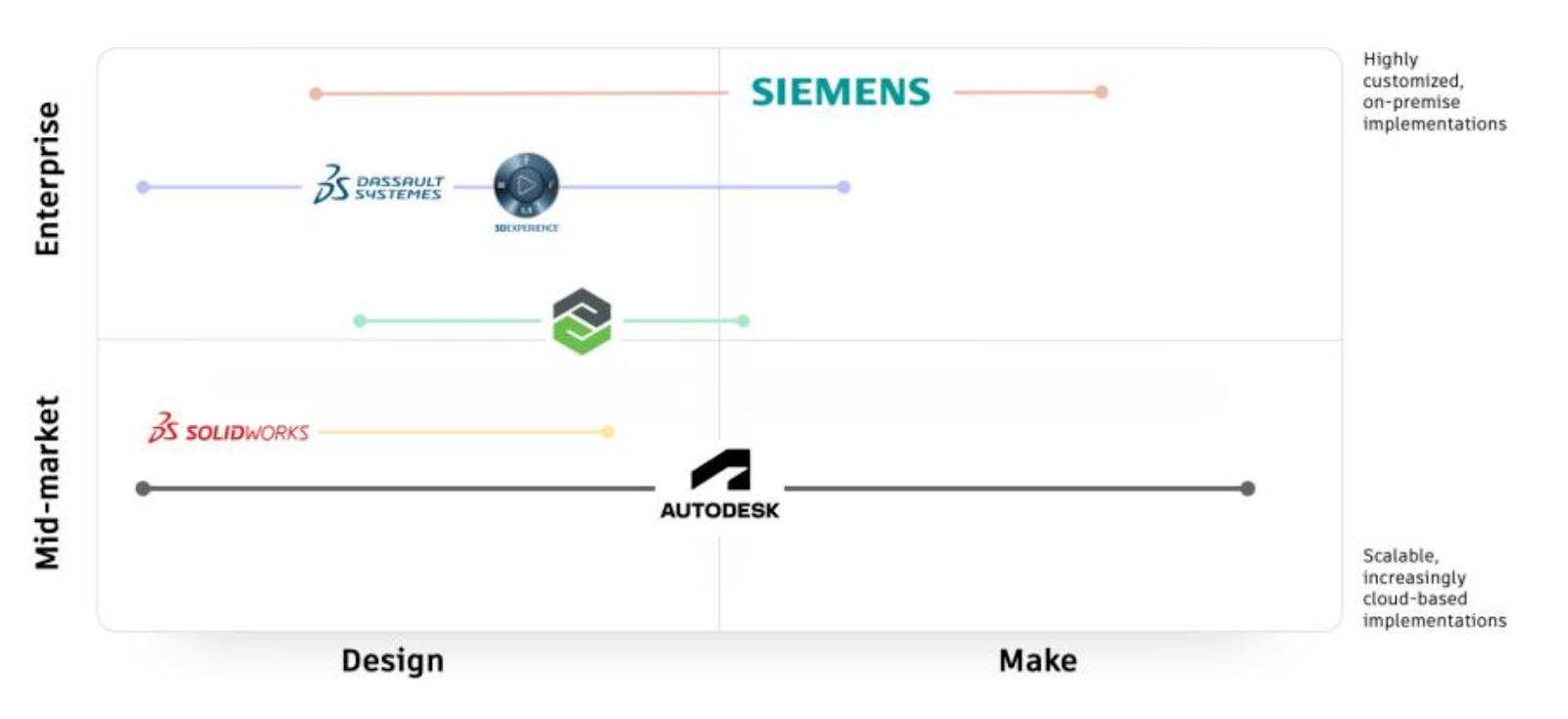
‍
Domain-specific CAD software
The best example of this is EDA software for chip design, with Synopsys and Cadence, two of the highest-valued software companies in the world. While many investors lament the cost of new chip design growing exponentially, we’ve seen that the cost of chip design increases have slowed as performance gains have as well. One of the more exciting trends in chip design is the verticalization of semis, chiplets, and packaging. As we’ve continued on a fabless model, we are seeing more organizations demand custom chips. While AI and automation have been used in chip design software for years (i.e., placement and routing), we think the incumbents have massive data advantages here, and startups will need to be very creative on their data positioning to avoid getting into IP issues.Â
Here’s a breakdown of EDA product segments and market leaders in each segment:
‍
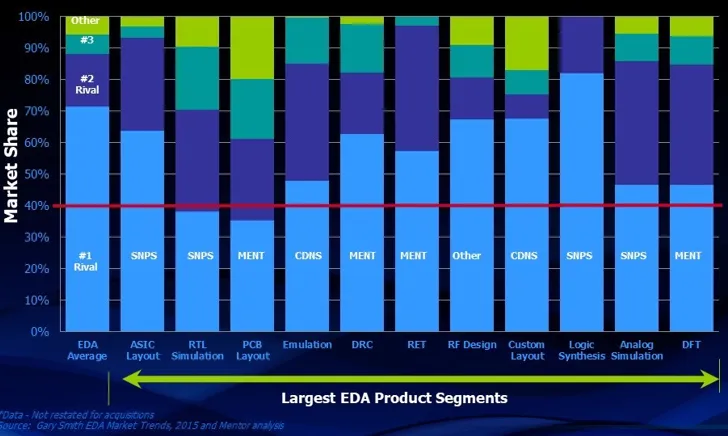
‍
Another example is infrastructure engineering tools for roads, bridges, tunnels, and plants. Bentley Systems is the market leader in this space with OpenRoad, OpenBridge, and OpenRail for designing their respective projects. They are essentially a standard in this space (supposedly, 48 of 50 US states use Bentley products). In plant design, Hexagon, Schneider Electric, and Bentley offer solutions for these products.Â
‍
Challengers
‍
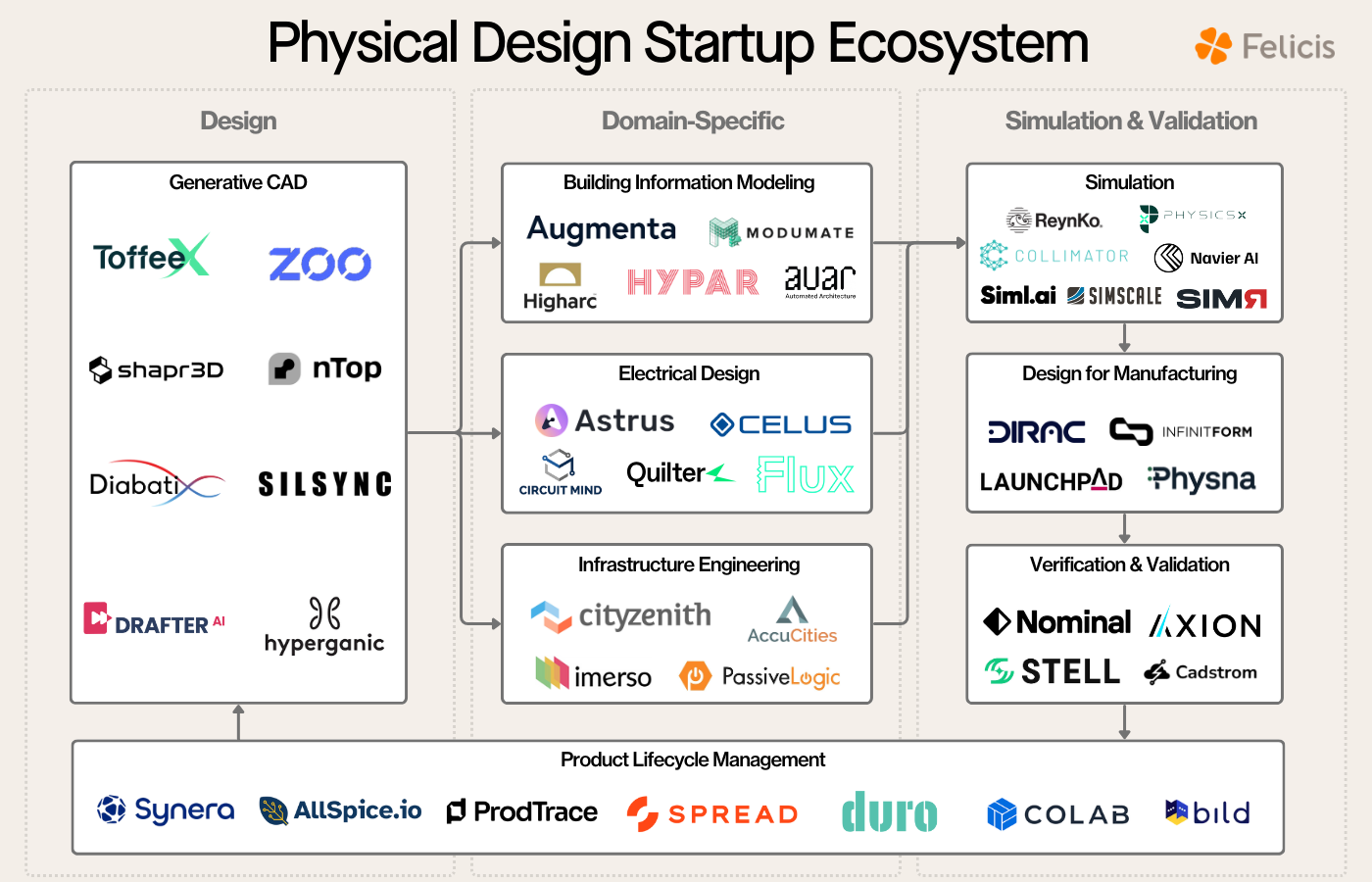
‍
No startup has broken into the upper echelon of CAD companies (yet), and relatively few have been acquired at $1B+ valuations.Â
One of the most successful startups came from the founder of SolidWorks, who founded OnShape in 2012, and sold to PTC in 2019 for $470M. Startups are attempting to take on each vertical in this space, but none have reached unicorn status.
The moats of the incumbents are so deep and the switching costs have such a high risk that this market has been mostly impenetrable. Hardware organizations are risk averse, with low novel tech absorption rates so we don’t think gimmicky “gen AI” features will scale. History has shown that it's difficult to build a venture scalable company if you don’t focus on mid market and enterprise. Still, we believe there are specific promising verticals for companies to pursue.
‍
What does the future of physical design software look like?
The future of these tools can be summarized in one sentence: “Cloud-based, collaborative tooling with an increasing amount of design automation.”
Industrial design tools like CAD are mission-critical software to all physical industries - from Cadence’s $78B business in enabling chip design and Dassault’s $50B business in mechanical parts design to Autodesk’s $54B business with construction modeling tools. While these tools garnered some of the highest enterprise ACVs, over the last 50 years, they have looked more or less the same.Â
‍
We are in the early stages of a paradigm shift in how we design and test hardware and complex physical systems. Specifically, there are four trends we’re excited about in this space that will see innovation in the next few years.Â
- Generative design. The most exciting trend in design software is generative design, an idea with a lot of potential but little value creation YET. As topology optimization (generative design) and simulation get better and faster, we expect more engineers will start to auto-optimize their parts and assemblies. Autodesk has trained a foundation model on 3D data called Bernini. While this (probably) will never get to the point where you say, “Design me a car,” there’s no reason why simple brackets, nails, or individual components won’t be able to be designed using generative models. There’s a real opportunity for a highly technical team to build exciting software here and help push the industry forward.
- Simulation. The gap from real to sim is closing and AI-powered simulations are one of the most interesting research trends. AI models could enable engineering teams to explore design spaces faster, deeper, and broader than possible with traditional PDE solver methods. Co-pilot capabilities could also change sim workflows—automating setup, cleaning up geometries etc. The bar here is very high and engineers have low trust after years of broken promises (i.e. bad results when test geometries are different from training sets) but we expect results and workflows to get better over time. Even the opportunity to just upscale turbulence data would be immensely impactful to hardware teams.
- Design for manufacturing. The other area that needs innovation is design for manufacturing (or constructability reviews in construction). This process requires taking a design, examining manufacturing processes and material availability, and deciding if it can actually be manufactured effectively. Digitalization of the supply chain will improve design integration so we can determine manufacturability earlier and earlier. As additive manufacturing continues to scale, new DFM platforms will become increasingly important.Â
- Automated physical design QA. Right now, AI is good at summarizing large amounts of data, taking context from requests, and using that to automate repetitive processes. It should be able to automatically assess designs, identify potential issues using data from manufacturing processes and material availability, and make optimization recommendations based on that.Â
Physical design is a complex space that provides a wonderful case study on what moats can look like in software. With increasing automation and verticalization, many long-overdue innovations are coming to this field. If you’re building in this area, please reach out to us.